Superconductors have already proven revolutionary in healthcare (they make MRIs possible) but could they help us achieve net zero? Prof Susie Speller says yes.
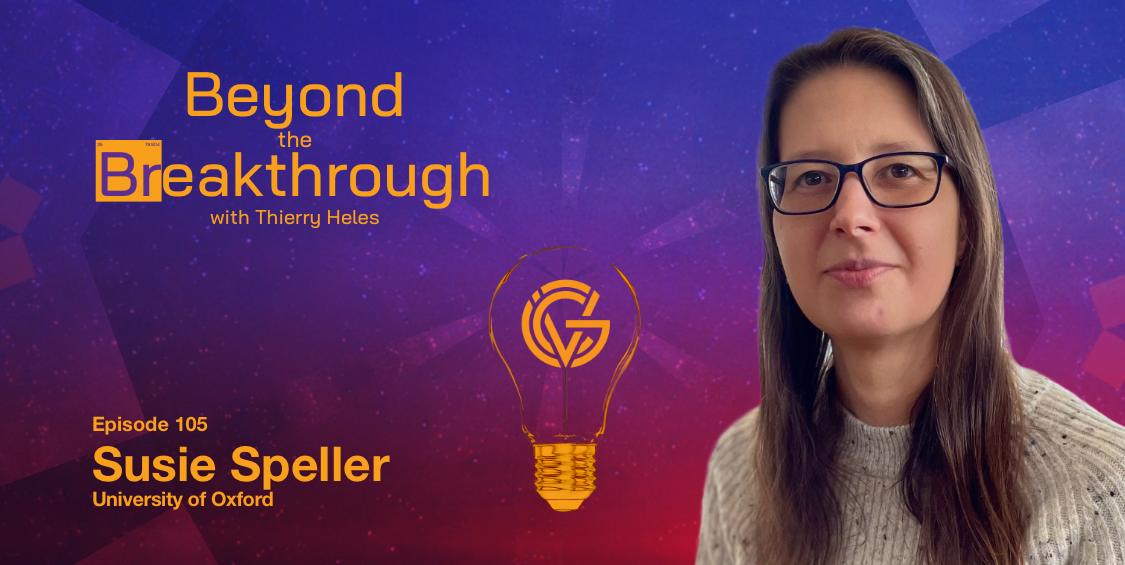
Superconductors can seem like magic if you watch magnetic levitation videos, but they’re far from a futuristic concept. They already have real-world applications: they are an essential component of MRI scanners, for example, which would otherwise take the energy of a small city to run (in reality, they take about as much as boiling a kettle).
Superconductors are also used in the development of fusion energy (they generate magnetic fields strong enough to contain the chemical reaction), they’re an integral part of the Large Hadron Collider, they’re being used in some approaches to quantum computing, and they’re being explored as transmission lines for the electricity grid and as a way to make offshore wind turbines smaller.
They’re not necessarily intuitive: superconductors must have defects to work — you might know that semiconductors are the opposite: they need to be (almost) pure. They’re also not without challenges — MRI scanners continue to rely on lead because no alternative has been found yet and figuring out how to create kilometre-long cables that could serve as power lines has taken three decades.
Prof Susie Speller is a fellow at St Catherine’s College and researcher in the Oxford Centre for Applied Superconductivity at the University of Oxford, where she helps corporates like Siemens Healthineers (which manufactures MRI scanners), fusion energy developer Tokamak Energy and scientific instruments company Oxford Instruments solve challenges around superconductors.
She is also a passionate science communicator and last year published a book on superconductors, A Materials Science Guide to Superconductors, that offers an accessible entry point into the field without glossing over the fundamental science behind it all. We find out what prompted her to write the book and how her interest in materials science began with a trip to the University of Cambridge when she was 15.
Global University Venturing chose to purchase a copy of Prof Speller’s book.
Subscribe
Transcript
Please note that the intro and outro have been omitted.
Susie, welcome.
Hello, nice to be here.
I look forward to a conversation. I’ve already read your book so I know a little bit about what you do, but I look forward to delving in a little bit more. As I said, you’re a professor in materials science, you focus specifically on superconductors.
To set the scene a little bit, can you give us an overview of what superconductors actually are and why they are so special?
Yes, of course. So superconductors are a particular class of material that have very, very special properties, electrical and magnetic properties. But their best property, I guess, that they’re most known for is that they have zero electrical resistance. Provided you cool these materials down to cold enough temperatures, their resistance goes to absolutely zero, which means that you can carry current without losing any of the energy that you’re carrying. So you can carry electricity from one place to another without losing any of it on the way. And that’s quite a big thing because normally we carry electricity with things like copper, which is a normal metal, and copper, we may lose about 11% of our electricity just getting to around the other side with those copper cables. So superconductors could potentially save us quite a lot of energy that way.
That’s quite a lot, 11%. That’s probably also contributing to carbon footprint quite a bit then.
Yes, yeah, that’s right. It’s one of the potential applications for superconductors is to try to replace those sort of transmission wires with superconducting versions that would be a lot more energy efficient.
We’ll delve into the applications a little bit more in a little bit. But I wanted to ask you as well, how did you become interested in materials science and what attracted you to superconductors specifically?
Yeah, so materials science is a subject that looks at why materials behave the way they do. So why does wood have the properties of wood? Why does a metal have the properties we know about it being a good electrical conductor, et cetera? And it turns out that a lot of those properties are concerned with, well, yes, the chemistry of what’s going on, what the atoms are and how they’re bonded together, but also structures on the microscopic scale. So not just the atoms, but how the materials behave on the microscopic scale very much affect how their properties are overall, their engineering properties. So that’s what material science is all about, really understanding why materials have the properties they do.
And I got interested in it to start with, when I did some work experience when I was at school. So I was 15, and I spent a week working in the engineering department at Cambridge University with the materials science group there. And I spent a fantastic week going out onto the fen and collecting bits of grass and other kinds of things, and coating them with gold and putting them in the electron microscope and seeing what they look like inside. And at the same time, I got to speak to lots of research students who were working in the department and find out what they were working on. That’s what sparked my interest in materials, this curiosity about why things behave the way they do.
And I used to go into that department every week to talk to the students all the way through my sort of studies in the sixth form, we call it, when I was sort of 17 and 18. And so decided that’s what I wanted to do. I wanted to do research in materials science. So that’s why I started getting interested in materials.
The superconductors, I started working on those when I was in the fourth year of my undergraduate programme. So it was a project year working on superconductors. And the reason I really took to them as it were, was because not only have they got absolutely remarkable properties, and we’re always interested in why things have the properties they have, they’re also very useful for lots of applications, so I could see where they could be used.
And finally, I’ve always been fascinated in what materials look like on the inside, and what we can do with electron microscopes to see what the materials are like on the inside. And superconductors have to have lots and lots of defects in them to make them work really well. So there’s always lots and lots to see when you put them in a microscope. So that was the final reason why I started working on them.
And they’ve captivated me ever since .I’ve worked on them for over 20 years now, and have loved working with this class of material.
That’s amazing. That’s really an argument for exposing children to science at a level that might be further advanced than what they would be studying in school as well.
It was an amazing opportunity for me to really just see what people were doing in research in science, and you know, what kind of questions they were asking and how they were using the techniques to look at answering those questions.
Yeah.
It really captivated me.
Something that really struck me, what you just said, and what also struck me when I read the book was that superconductors need defects. Was there something that you found surprising about superconductors when you first started this research?
Well, in some ways, yes. And that’s because before you learn about superconductors at university, you learn about semiconductors, certainly as a materials scientist. And semiconductors are basically silicon. And to make semiconductors work really well, they need to be almost completely pure silicon with as few defects in it as possible. So they’re single crystals. In fact, even the surface of it is a problem. The fact it’s got a surface is a problem. You do put some impurities in there deliberately, but in the parts per billion level. Superconductors, I mean, people get the two confused quite easily, but superconductors are completely different. To make them work and carry really large currents, which is what they’re very, very good at, you have to put in lots and lots of defects. So it’s surprising from that point of view, because of the other electrical materials I was familiar with, metals as well, their conductivity gets worse with impurities. So it was a bit counter to those other materials that we’re used to.
But as a materials scientist, I’m quite used to materials getting better with defects in lots of other ways. So for example, steel is essentially mainly iron, but steel is much stronger than iron. And the reason is because we put a small amount of carbon in there as an impurity, and that carbon goes into the spaces between the iron atoms and interacts with the material and the defects actually inside the material that move around when a material deforms. So they get in the way of the defects that naturally occur in the material that make it deform plastically. And so you can strengthen it by putting that carbon in there.
There’s lots and lots of examples like that in material science, where, for example, you might make the size of the crystals inside your material much smaller, because the boundaries between crystals are defects, and they interact with the way in which the material deforms, for example.
There’s many, many examples in materials science where you deliberately want to engineer the material to have defects in there to make its properties better.
That’s really fascinating, actually. I liked… it was an example of, you could make a spring out of concrete, if you just knew where to place the defects, which was really quite a strange idea. Because you always think of concrete as something that’s not that flexible, really.
That’s right. So I mean, it’s a brittle material, like glass. And in fact, with brittle materials, what tends to affect how strong they are, how they behave, is the maximum size of the crack inside them. So if you make the material without any cracks in, then they won’t behave in the way we’re used to seeing them behave, they won’t deform in the same way. They’ll have very different macroscopic properties than if they’ve naturally got the cracks that we’re used to them having in there.
I’ve alluded to it a few times now, you published a book, it’s called A Materials Science Guide to Superconductors. I will put a link in the show notes as well, so people can find that easily. How did you become interested in science communication?
I think this is something I’ve naturally been interested in all the way through my career. And partly, it comes from, I do a lot of teaching. So I teach undergraduates a lot. And from that, I see the students coming through. And I can see that we need to do things like increase the diversity of our student cohort. So in the physical sciences, for example, we’re underrepresented by women, also by lots of other groups. And one of the things that I’ve always wanted to do is to go out and show schoolchildren, for example, that there are women doing physics and subjects like engineering and materials science. It’s not something that isn’t for girls. I’ve always taken the sort of outreach side of things very seriously.
But I also just love talking about what I do. I love talking about superconductors. I think superconductors are remarkable materials, and absolutely fascinate me. And I want to fascinate other people with them, is what really, you know, leads to me wanting to communicate that more widely than just the other people who are also interested in superconductors. Of course, we do that communication too, in the form of conference talks and publications that we write. But being able to communicate that science to the more general audience, why we’re doing it, why it’s interesting, inspiring other people, makes you feel so good. And it’s so enjoyable to do that I really love doing that.
And my book came about really, because there’s not very many publications on materials science for the sort of age group of sort of pre-university. So those students who are looking at science at university, and want to get some information about what’s out there. So what I really did was I took my third year university course on superconducting materials, and flipped it round to explain some of the materials science that was needed to understand the superconductors through those examples of superconductors, instead of what I do in my degree programme, where they learn all about lots of materials science. And then I say, well, this is how it applies to superconductors. So I kind of flipped it around and made it more accessible for a more general audience.
You’ve definitely succeeded in that. I’m a little bit older now than a 15 year old. But it is totally also the kind of book that I would have enjoyed reading before I went to university. It’s very, I say very accessible. There were a few chapters in there where I struggled a little bit. My quantum mechanics knowledge is rusty.
Yeah.
But it was fascinating.
Another thing that I thought about is that a lot of science books aimed at this audience are quite dumbed down. And so don’t have any of the technical stuff in there, just so that they can make it completely everything can be followed. So I deliberately tried to make sure I included some meat in the discussion and not try to dumb it down completely. Because I think that certainly students who are going on to want to study these kinds of subjects like being challenged and like having to think about what they’re reading, not just understand it all as they read it the first time. So it’s deliberate that there are little bits in there that are a bit more challenging, yeah.
It’s a book that I will probably reread. Can you tell me a little bit about the work that you do with the industrial partners at your Oxford Centre for Applied Superconductivity?
Yes, of course. So the Centre for Applied Superconductivity was set up a number of years ago now in Oxford with that real aim to work with the local industry in superconductors, of which there are a lot around Oxfordshire. We have a number of different companies who make superconducting magnets, for example, or use superconductors in a variety of ways. And what we found through our interactions with them was that there’s quite a lot of problems that they didn’t have the R&D capability or the expertise to tackle themselves.
So it’s sort of pre-competitive research, but in topics that they need to get answers to, to develop their business. And this is really amazing for scientists to be able to work so closely with industry, because what we want to do is to make our science applicable to the real world and to get our science and what we’re researching actually out there and into products. So if we’re working on problems that industry really have and really want to know answers to in collaboration with them, then we can feel that we’re really making an impact beyond just understanding what’s going on with atoms arranged in particular ways. So that’s what we try to do. And we do that in a number of ways, including having joint students. So our industrial partners quite often will partly fund our research students so that we can do these projects, which have a focus on what the industry partner really, really needs, but also have intellectual interest for us. So there’s interesting materials science to do.
You said pre-competitive research. Have there any kind of applications that have come out of it that you are able to talk about?
So the kind of thing we’ve been looking at, and that hopefully will make it into products that are being designed and developed at the moment. One of the areas that we’re working on are superconducting joints, we may touch on it at some point, but machines like magnetic resonance imaging magnets have superconductors in them. And these particular magnets need to have joints between lengths of wire that are truly properly superconducting. So we can’t have a break in the superconducting circuit for this kind of magnet. And the industry who make MRI magnets, they know how to make them for these joints, for the conventional materials that they use.
There are a few problems with them. Sometimes the materials they use, sometimes because we want to start to introduce newer superconductors, different kinds of superconductors into these products, and there isn’t a mature technology yet for joining them. So one of the things that we’ve been working on with Oxford Instruments and Siemens Magnet Technology, or they’re now called Siemens Healthineers, which are both based in Oxfordshire, is on these joints between different kinds of superconducting materials. And that’s a real materials science problem that we’re very well set up to investigate. And the industry themselves haven’t got the capabilities to do that fundamental research on how we can make better joints or new joints between new classes of superconductor.
So that’s one of the areas that I’m working on. Another one with industrial partners is in the area of radiation damage of superconductors. So that might sound very complicated, but superconductors are an enabling technology for nuclear fusion reactors. I mean, there’s been a lot about nuclear fusion in the news recently. So one particular kind of fusion reactor that use magnetic fields for the confinement needs superconductors. And so we’re working with companies that are interested in fusion. So not only there’s the UK Atomic Energy Authority, who deliver the sort of government programme on fusion, but there’s also private companies like Tokamak Energy, who are building fusion machines using superconductors. And one of the questions we have is, how long will the superconductor last in the environment of a fusion reactor? I mean, fusion reactions are basically what happens in the sun. So in a fusion reactor, you’ve got a sun confined within a device on earth, and your magnets are quite close to that sun. And lots of particles are generated that can damage your superconductor. So we’ve been doing research that’s been looking at that kind of damage that’s introduced in the superconductor. And again, that’s something that our partners aren’t really very well placed to look at. It needs very high end materials characterisation techniques, for example. But we’re quite well set up to take on those kinds of challenges to try to find out what’s going on, how long will the magnets last and that kind of thing.
That’s really cool.
That’s a couple of examples of what we’re doing at the moment.
We’ll get back to MRIs because I want to talk a little bit more about those in a minute. But I want to stay on your research for a second. You also research the fundamental properties of novel superconductors, Fe-pnictides and chalcogenides. Can you tell me a little bit more about this work?
Yeah. So this is a little bit different to my work, which is linked to industry. There was a class of superconductors that were discovered in 2008 called the iron-based superconductors. So quite a recent family. And these actually have the second highest superconducting transition temperature. That means that they, out of all the kinds of superconductors that there are, this family of materials superconducts at really quite high temperatures compared to a lot of the others. When they first were discovered, there were lots of interesting physics that was noticed.
So what normally happens is that people first of all synthesise some bits of powder and discover that there’s a superconductor there. And then they try to make things as single crystals, as pure as they can, as perfect as they can as single crystals. And they do lots of tests on those to try to figure out the fundamental physical properties of the superconductor that they’ve made. So these crystals had been made and lots of tests had been done using synchrotrons and all sorts of expensive bits of kit.
One of the questions was, well, we can see both magnetism and superconductivity at the same time. What’s going on? Have we got a material that’s both a magnet and a superconductor concurrently, or has it split into two different materials? One that’s a superconductor and one that’s a magnet. And you might think that’s quite easy to find out, but it isn’t that easy. But this is perfect materials science because that’s what we look at. We look at multiple things going on within a volume of material. We use microscopic techniques, you know, microscopy to look at what’s going on. And so I applied some of the techniques that I use in other areas of my research to look at this separating into different types of material that goes on within these crystals. And we found, I’m not saying that all kinds of iron-based superconductors have this, but several of the ones that we looked at definitely split into different things, even though they look, when you do the sort of bulk assessment of the single crystals, it looks to be one thing. Actually, it’s not. I use quite a lot of different microscopic and spectroscopic techniques to really get to the bottom of what was going on in some of these crystal systems, which is very interesting.
Yeah.
We answered some of the questions that the fundamental physicists had about the sort of emergent physics in this new class of superconductor.
That is really cool. If you ever write a book about that, I will definitely read that as well.
People who’ve been around for a little while might remember that TIME proclaimed the superconductivity revolution on its cover all the way back in May 1987. It’s safe to say that may have been a little bit premature. Why has it taken a few more decades for the technology to become usable and now to be on this cusp of it potentially changing so many industries?
That’s a really good question. The reason that the superconductivity revolution was a huge big thing, this article in TIME, but also a conference that was known as the Woodstock of Physics, that happened at the same time. This is because they discovered a brand new class of superconductor that has superconducting transition temperature of above the temperature of liquid nitrogen. Liquid nitrogen boils at 77K, which is 77 degrees above absolute zero, about -200°C. It might seem very cold still, but it was a major breakthrough that these materials could work and superconduct using liquid nitrogen as a cryogen. So something you could cool it with liquid nitrogen. Liquid nitrogen costs about the same as milk. So it’s really quite a cheap cryogen. Whereas the previous materials that had been known about superconductors, that had been known about needed usually liquid helium to cool them. Liquid helium boils at 4K, so four degrees above absolute zero. And that was much, much more expensive and much more limiting in terms of engineering of devices using liquid helium.
So that’s why in the 1980s, late 1980s, when this class of materials were discovered, they thought it was going to solve all our problems, all our energy problems, because nitrogen was so cheap, so easy that we could just make all our transmission cables straight away out of these new class of high-temperature superconductors as they’re known.
Turns out it wasn’t as simple as all that. And the reason comes down to the material science. So these materials, they’re made out of, well, various different things, but one of them that’s the sort of archetypal material is called yttrium barium copper oxide. So it’s got four different things in, although quite a lot of them have five or six different elements in, but they’re an oxide. Okay. And oxides are ceramic materials. So they have the mechanical properties of something like a teacup. And if you want to make say a transmission cable out of it, then you need multiple kilometres of something that you can bend and wind and make it a wire. And ceramics aren’t well known for their ability to be made into wires very easily because of brittle nature of the material.
So that wasn’t very helpful just to start with that they were going to be much more difficult to make into wires, but there are other problems with them. They’re very chemically sensitive, which means that they have a certain ratio of each of those different elements that you need to have in the compound. And if you get it just slightly wrong, then it won’t superconduct anymore or it will lose some of its superconducting properties. It won’t be as good. So you have to get everything, including the oxygen content spot on and oxygen happens to move in and out of these materials very easily. So it’s not straightforward to get them chemically just right. And they react with metals. So if you are processing a ceramic, you normally need to process it at 700, 800, 900 degrees C. If you had one of these superconductors in contact with most metals at those temperatures, they would react and form something else and you wouldn’t have a superconductor anymore. So all of these things were problematic. The only metal they don’t mind is silver, pretty much. And of course that’s pretty expensive.
The final problem with them in terms of the materials science was based on one of their fundamental properties, which means that if you’ve got many different crystals in your material, most materials are polycrystalline, so they have lots of different crystals in them. At the boundaries between those crystals, you have walls that are called grain boundaries. Those features which are where the structure isn’t quite perfect, where you’ve got two different crystals meeting each other, they’re enough to act as a dam to the current flow in these particular class of materials. So if you think of your current being like a river flowing, that’s the electrical current going through your material, these grain boundaries as we call them, the boundaries between crystals, act like a dam and stop the current flow.
So you have to make these materials in multiple kilometre lengths with no grain boundaries, so single crystals. That’s no mean feat. It’s a very difficult, challenging bit of material science. And the fact that we can do it is pretty remarkable. So now, 30 years on, we have some amazing materials that can do just that and can carry enormous currents. We’ve overcome the problems of their mechanical difficulties, the fact they’re ceramics. We’ve overcome the chemical interaction problems, and we can make them as pseudo-single crystals a kilometre long. So we have materials, they can be wound into magnets, they can be wound into transmission cables, they can be cooled with liquid nitrogen, but it has taken a long time to get to that point.
In fact, 30 years is a normal kind of timescale for materials development from discovery of a new material to having it in a form that it can be used.
Listening to you, it almost sounds like, as a layperson, it sounds very fast when you lay out all the problems. I’m like, “Oh, only 30 years. That’s kind of remarkable.”
Yeah, it is pretty remarkable, actually. Yeah, these really quite major challenges can be overcome. And it’s for those reasons that challenges like fusion, which seem to have huge, huge engineering challenges on all levels, scientists are still optimistic that we’ll solve them, because we can do remarkable things. We’ve shown that we can do remarkable things. So yes, there are challenges, but that’s what drives us on, actually.
Yeah, that’s very true, actually. It would be very boring if we didn’t have any problems to solve. As you mentioned, superconductors are used in MRIs, they’re also used in the Large Hadron Collider, they’re used in maglev trains, quantum computers, fusion energy. Is there an application where you think it will be particularly revolutionary? And is that an application that is close, or is it something that is still really far away?
So I think it can actually be revolutionary in quite a lot of areas. It already has been for healthcare with MRI, which would not have been possible without superconductors. Fusion, for example, will not be possible… Commercial fusion, so making power plants that will give energy to the grid, won’t be possible without superconductors. So it’s an enabling technology for that.
Other areas where I think it’s got the potential in the really relatively short term are things like power transmission cables, which now we’ve got the material. The main problem there isn’t the properties of the material or the technology or the engineering of the cooling or any of those kinds of things that people might think are the problem. The problem at the moment is the material is too expensive. Because as I’ve said, you have to make it in very clever ways in order to cope with the fact that it’s a ceramic and all of these kinds of things. So it’s too expensive at the moment.
But technologies like fusion that require this material, and they haven’t got any option, will increase the volume that’s being produced, will scale up the material production, and that will bring down the costs. There have been lots of predictions about how much the costs are able to come down with this scale up. And now we have this driver that has to have this material. So this volume scale up will be happening over the next few years. So the material should be cheap enough with any luck, hopefully, that we can see it in all these power applications.
So transmission lines, like I said, but also wind generators, the generators, the electrical generators that go into wind turbines, you could make great savings by using superconductors there, not necessarily just on efficiency, but on size and weight, which makes a huge difference to cost of things like offshore wind turbines, if you can make them a lot smaller and lighter, that’s a really good thing. So there’s lots of avenues where superconductors have the potential in the next sort of 10 years or so to make a difference. Fusion has always been thought to be 40 years away. But there’s been a big change recently in private investment in fusion, which is really accelerating development. And there’s incredibly ambitious timelines for fusion, which are much shorter than the original roadmaps were for when we would have the first commercial fusion reactors. So there’s promise there that even those won’t be on the sort of snail’s pace for adoption.
We’re at a time with superconductors now, where the materials are really there and have been demonstrated in lots of technologies, actually, and we’re ready to use them. It’s about getting them into these applications, about persuading power companies that superconductors can save us energy, and can contribute to net zero, our targets for net zero. They’re one of the few really revolutionary technologies that can do that for us. And they’re ready to be used now. So huge promise.
Yeah, I want to pick up on your mention of power lines as well. Something that kind of struck me from your book as well was the discussion of AC loss. Can you talk a little bit about that and why that makes it a bit more of a challenge?
Yes. So power lines at the moment work under alternating current conditions. So you don’t have steady current going along the wire. It’s fluctuating backwards and forwards and alternating. There’s lots of good reasons for that, about why that’s what we’ve got in this country and pretty much I think every country. Superconductors have this zero resistance property, but they’re only actually truly zero resistance when they’re under direct current, so steady current, rather than under alternating current. There’s various bits of physics that contribute to that, one of which is to do with how particular defects that are created in the material when they’re in a magnetic field, how they move backwards and forwards, and the fact that they dissipate energy when they do so.
So there’s various reasons that you get this AC loss. You also get what we call coupling losses, which is to do with the fact that we have multiple filaments within a superconducting wire and we have multiple wires making up a cable and currents can cross between them. And when they do, they have to go through non-superconducting stuff and that generates some resistance.
So there’s various different physics that contribute to AC loss and there’s lots of tricks that we can play when we’re designing the wires in order to try to minimise those losses. So we do things like making each filament, so each little strand that carries the current, we make them very tiny and we have lots of them. And by doing that, we reduce what we call the hysteresis loss. To reduce the coupling losses, we twist those wires and we weave cables to move wires from one place to another and all of those things help us to reduce AC loss.
So we kind of design the components to try to minimise that loss. But it is there. One of the things that we could do with superconductors, if we’re going to replace transmission cables, long distance transmission cables with superconducting ones, then we would probably want to use DC cables. Superconductors are amazing under DC and actually long distance transmission cables, there’s talk of going to DC cables anyway. It’s called high voltage DC, HVDC. And with a superconductor, we could do DC without the high voltage. We have no resistance, we don’t need that high voltage, so they would be a great technology. But it means that it doesn’t match up with our existing infrastructure, which deals with AC. So if we’re going to move to superconducting technology and the best superconducting technologies overall for our power, then we would probably be wanting to change various elements of how our network and distribution and electrical grid work.
Thinking of the grid, we’ve got a lot of capacity problems coming up with our grid. In the UK, it’s called the National Grid because of the drive to using things like electric vehicles and electrification of all sorts of different things means that we’ve got to get more electricity from the power stations to where it’s being used, from the renewables, like the wind turbines and the solar farms to where it’s being used. And we’re looking at a three times increase in capacity that we need for our grid. And so superconducting technologies are one way of doing that, because they carry much more current actually for the same sort of size of cable. And they would have to be underground cables, but they would not need so much right of way clearance as you need with pylons and things like that. There’s lots of advantages, environmental advantages to going to superconducting cables, not only the efficiency savings that we could make.
Yeah, that’s kind of amazing. Three times. I kind of understood that there would be more demand on electricity, whether it is EVs or even things like heat pumps, replacing boilers, but threefold, that is a lot. That is really quite a lot.
Yeah. There are predictions that say that threefold increase. I’m not sure over what timescale, but yes, it’s a big, big problem, a real problem.
I do want to take a closer look at MRIs, as I said, mostly because I think that’s an application that most people will know. I don’t think a lot of people actually know how they work beyond the fact that they have a magnetic field in them. Can you tell me a little bit about why superconductors are so crucial in them and could they possibly exist without them or are they absolutely necessary?
The MRI machine, one of the main components is the magnet that produces what we call a static and uniform background field. So this is a constant, stable magnetic field that’s the same across the whole body. When you see an MRI machine, you’ll see a big sort of cylinder, essentially ring that you get wheeled inside when you have the scan. That thing that you’re being wheeled inside is a superconducting magnet. The superconducting magnet is providing this stable, static, uniform background field that’s absolutely essential for MRI. The way it works, if you want a little bit of physics, is that it causes water molecules in our bodies, hydrogen in there, to resonate, which means it sort of vibrates in a particular way. And that happens at particular magnetic fields.
And the way we do the imaging with it is by, on top of this background field that’s very uniform and stable, we have a gradient of magnetic field on top of that, which means that there’s only one place inside the body that has the particular magnetic field at any one time that’s causing these molecules inside your body to resonate. And that’s where you’re getting the signal from. Changing that gradient, you can image across the body, but it means you have to have this really stable background field that doesn’t change. Otherwise it makes all of the processing of the data much more difficult. Otherwise you don’t know from where in the body the information’s coming from. So the job of the superconducting magnet is to make this really stable background field.
For a superconductor, it’s not a difficult, a high magnetic field to create. It’s a really relatively low magnetic field. So we’re not pushing the capabilities of the superconductor terribly hard. The main thing about these magnets is twofold. One is that they are much more efficient than they would be if you had to use a resistive magnet, so like copper. And the reason for that is that they’re a complete superconducting circuit. So it would surprise people to find out that these magnets are shipped from the factories where they’re built — one of which is just outside Oxford — they’re shipped from there with all of their liquid helium. They run with liquid helium, these magnets. They’re shipped with their liquid helium already in when they leave the factories. When they’re installed in the hospital, they have a power supply to start the current going in the magnet, and then they close a special switch and take the current supply away. So there is no power supply to the magnet when it’s working in the hospital. So they operate without a power supply. So they don’t require large power supplies to keep them going.
They don’t use all that energy that you’d need to use if you didn’t have a superconducting magnet, because they’re a complete closed superconducting circuit. Once you start the current in there, the current will go on forever. And when I mean forever, the decay time is something like 10,000 years, one of these magnets, because they’re truly zero resistance, right? So even though the joints in there aren’t absolutely perfect, so they introduce the tiniest, tiniest bit of resistance, they still got this incredible ability to keep their current and keep the magnetic field stable. So that is the reason why we need superconductors. One is because they don’t need the power supply. So we don’t use a lot of energy to keep them going. In fact, we use about the power of a kettle, which is enough to recondense any helium that boils off, because we don’t use any heat in there. It’s not boiling off helium at a fast rate at all. It’s very slow. So you can just use this mechanical thing, a bit like a fridge, to recondense that helium and keep it in there.
And the second reason is that because this decay of the magnetic field is so slow, 10,000 years, it’s completely constant. It doesn’t change in time. So it’s perfect for MRI. It’s got this perfect, stable background field that’s what’s needed. That’s the two reasons why superconductors are really necessary. To power a magnet of that size, which wasn’t superconducting, you’d probably need the power from a small city.
Wow.
Rather than a kettle. I mean, that’s the difference it makes.
10,000 years. I mean, that’s almost a perpetual motion machine, if such a thing existed.
Yeah, well, I mean, if you think about it, for people who know anything about atoms and electrons and orbits in atoms, those electrons stay in their orbits indefinitely. It’s a quantum phenomenon. There is no decay of that. Same is true with superconductors, like a big atom. The currents don’t decay. It’s a macroscopic quantum phenomenon. So it behaves like an atom on a giant scale.
You’ve alluded to this before, I think, the joints in MRIs. MRIs are still allowed to contain lead. There are exemptions, I think they’re set to expire in 2027. Why is lead so important? And what is a possible replacement for when this exemption ends?
MRI companies are worried because their technology for making joints, these are the perfect links between bits of superconducting wire, uses lead. And lead solders, so lead is a key part of solders that have been used traditionally for electrical components and plumber’s solders, all of those contained lead until fairly recently. It’s a low melting point metal and it forms alloys that have very low melting points. So that’s why it’s good as a solder, which means that it’s good for joining things because you can melt it and not melt what you’re joining.
Industry like the aerospace industry that used to use a lot of lead-based solder, they’re not allowed to anymore because there are alternative materials they’ve had to use instead. Problem is that lead is toxic. Probably people are aware of lead in paints and things in the old days, which no longer is allowed to be there, for example. So everybody wants to remove lead from all of the products.
MRI has this exemption. And the reason is that we haven’t got an alternative to lead solders for superconductors. And the reason for that is that all of these alternative solders that people have developed that are lead-free aren’t actually superconductors. So if we’re going to make a joint between two superconducting materials that is superconducting, the thing we make it with has to also be a good enough superconductor. And lead is, apart from niobium, which is probably the element that has the best superconducting properties, lead is the next best. Niobium has a very high melting point, so we’re not going to use that for a solder. But lead is so much better than anything else that the lead alloys that we can use, lead tin, lead bismuth, things like that, they have much better superconducting properties than any of the alternative solders that people have used for other applications.
So it’s just not possible to switch for a different solder. We have been working with the MRI companies to look at alternative jointing strategies. So maybe not using solders at all, maybe using some kind of welding or cold pressing or other techniques so that you’re not reliant on the property of that solder material to get your joints.
But you’ve got to remember that MRIs, I mean, Siemens ship about three MRI machines a day. All of the joints are handmade. And if any of those joints fail, their machines have to be shipped back to them. So it’s a huge embedded cost associated with any joint that goes wrong. So whatever technology we use to replace lead-based solders in MRI, it’s got to be incredibly reliable. About 10 joints per MRI machine, they’re making three a day in that one factory. So it’s got to be able to be made on that scale and with no failures. So that’s the difficulty we have.
And until… I mean, the hope is that until such a solution has been found and has been demonstrated, they will, with any luck, continue this exemption that MRI has this allowance to use lead in their joints. Because otherwise, they wouldn’t be able to make any more MRI magnets that would work the way they have done.
You’d think that MRIs being such a critical part of healthcare, the powers that be would be flexible. If we get to 2027, and there is no other solution.
We would hope so. So obviously, the companies that I work with, what they’re worried about is one of their competitors will find an alternative solution, and then they will be losing an advantage or their competitors will be gaining an advantage over them. So it’s a real problem for industry, and they’re working actively to try to solve it. And it’s some of the projects that I’ve had with Siemens, for example, have been on that.
One of the things that has been considered the holy grail is room temperature superconductors. There are some examples of it. Why aren’t we using them?
So the room temperature superconductors or near room temperature superconductors are what we call the super hydrides. And these have only actually been discovered in the last few years. They’re things like hydrogen sulfide, and then there’s a lanthanum hydride, things that have got a lot of hydrogen in them anyway. And some of the reports are quite close to room temperature.
The problem with them is to make them into a superconductor, you have to put them under enormously high pressure. So they have to have about, I think it’s off the top of my head, it’s something like 100 gigapascals. That maybe doesn’t mean much to everybody. It’s about, I think it’s about two thirds the pressure at the centre of the earth, that kind of number. Don’t quote me completely on that, but it’s that kind of number. These are enormously high pressures. And that’s much, much more difficult than cooling them down with liquid nitrogen.
So in terms of being technological material that could be used for magnets and cables and things like that, this enormous pressure that they need really means that they’re not useful for applications. They’re interesting in terms of understanding their superconductivity. So there’s a lot of theoretical and fundamental interest in these materials. But in terms of being used for real applications, they’re unlikely to yield any answers there.
Yeah, that makes sense. 100 gigapascal is almost unimaginably high. How do you even make that in a lab?
Well, they do it with diamond, it’s the strongest material in the world. And they have these things called diamond anvil cells, which essentially are diamonds that push a tiny bit of the superconducting material. I mean, they’re gases under ambient pressure, so they’re not even a real material as such until they get squished. But it makes experimenting on them very challenging as well, which means that, you know, there’s being absolutely certain you’ve got superconductivity, demonstrating the normal things that we have to demonstrate to show that we’ve got a superconductor becomes a lot more difficult when you’ve got to do it under these enormously high pressures.
And so some of the reports that have been mentioned, the ones that have mentioned real sort of room temperature, have actually been retracted, or are under review, because there’s been some concerns about them, about whether they’re real or not. But there are some that are definitely believed in this class of materials in these super hydrides.
Fascinating though. That is almost all the questions that I had. It’s also almost all the time that we had, sadly. Is there anything else that you wanted to say?
Not in particular, but just really to tell everybody that we’ve got remarkable materials, they are ready to be used. So now it’s just a case of getting them into the applications, convincing power companies that superconducting technologies where they need to cool with liquid nitrogen isn’t an impossibility.
And with any luck, these sort of developments will come in the next 10 years, and hopefully people will see superconductors being used a lot more around them, particularly to help with this transition to net zero, which is really important for all of us.
Susie, thank you so much for taking the time to chat with me. It’s been incredibly fascinating, and I’ve learned so much about something that I barely knew anything about before. Thank you so much for taking the time.
Oh, it’s my pleasure.
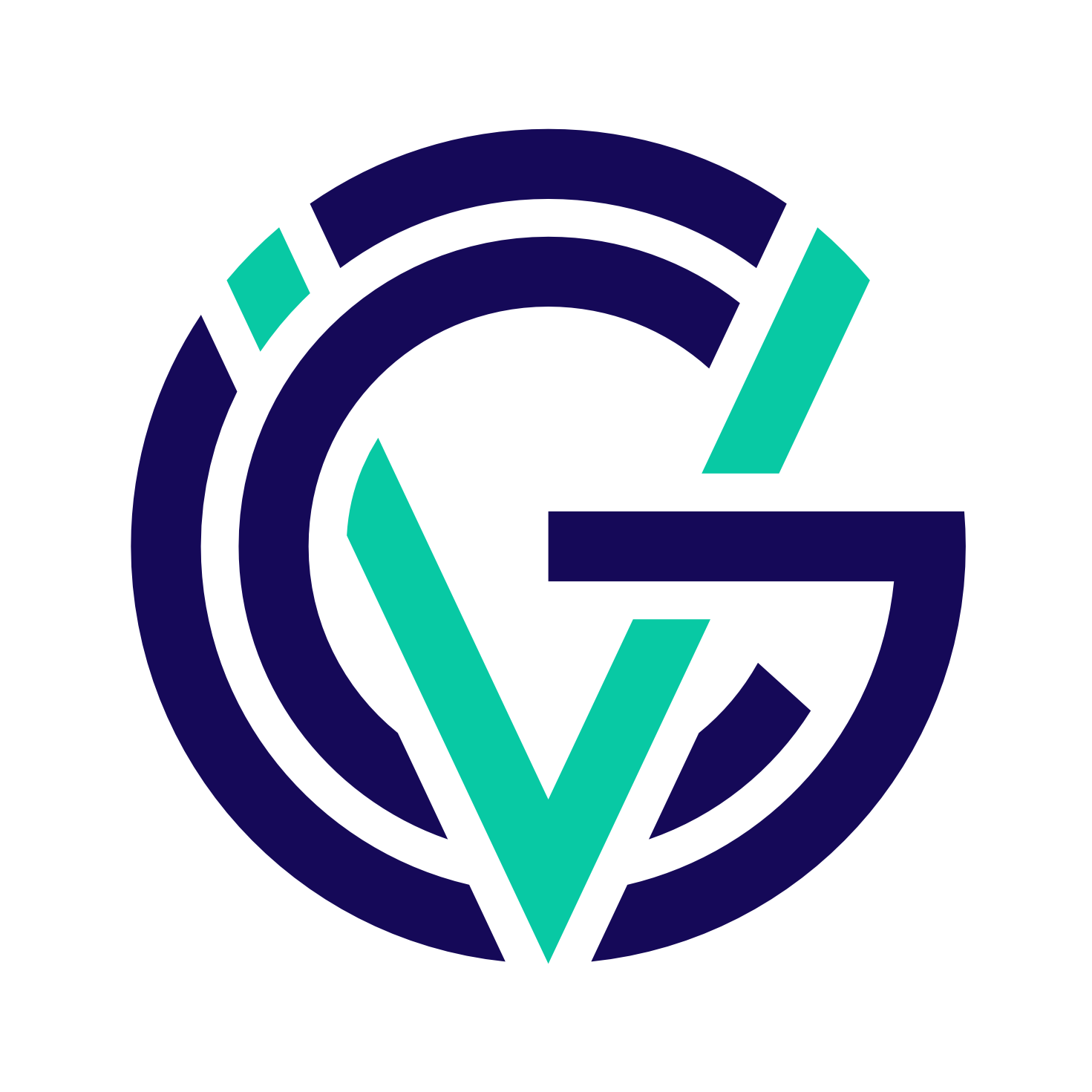
Thierry Heles
Thierry Heles is the editor of Global University Venturing, host of the Beyond the Breakthrough interview podcast and responsible for the monthly GUV Gazette (sign up here for free).