Not only are we not capturing enough carbon but we are not making the most of what is collected. Using it in plastics production is an area we should explore further.
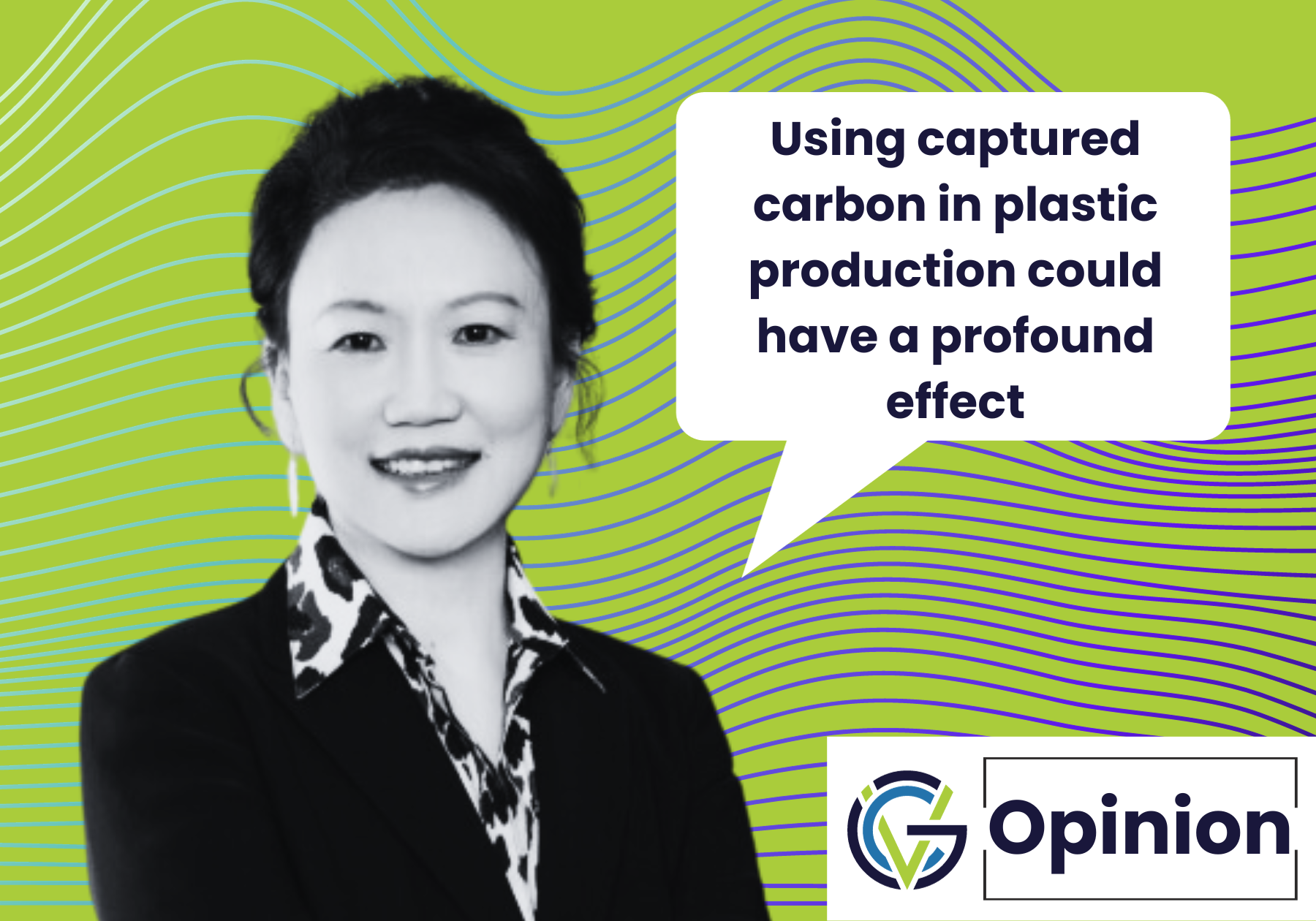
Carbon capture, utilisation and storage (CCUS) is one of the few frontline tools we have for fighting climate change. Today, carbon dioxide is captured directly at power generation and industrial facilities, or even directly from the atmosphere. The captured CO2 is used or stored. If stored, it’s injected into deep geological formations. If utilised, carbon can be incorporated in a number of production processes.
But not only is not enough carbon being captured, we are not making the most of what is collected. Carbon has the potential to be a useful, abundant and cheap raw material. But many of the processes to use it aren’t economically viable, or may simply re-release the carbon after a short time.
Using captured carbon in plastic production is, however, one of the most promising areas of usage that has developed in recent years.
Ramping up our ability to capture CO2
There are more than 30 large-scale facilities in operation or construction globally as of the end of 2020, according to official figures from bodies like the International Energy Agency (IEA). This is only a fraction of what is needed. An estimated 33 billion tons of CO2 are emitted each year, but in 2021 there was capacity to capture just 43 million tons. That is 0.1% of what needs to be done.
The target is for CCUS to reach 10 billion tons/year eventually, removing a third of our annual carbon emissions annually. But while capacities are increasing year by year (the next goalpost is 279 million tons of CO2captured/year by 2023, according to BloombergNEF’s report), not enough of that captured CO2 has been applied in innovative, practical or useful formats.
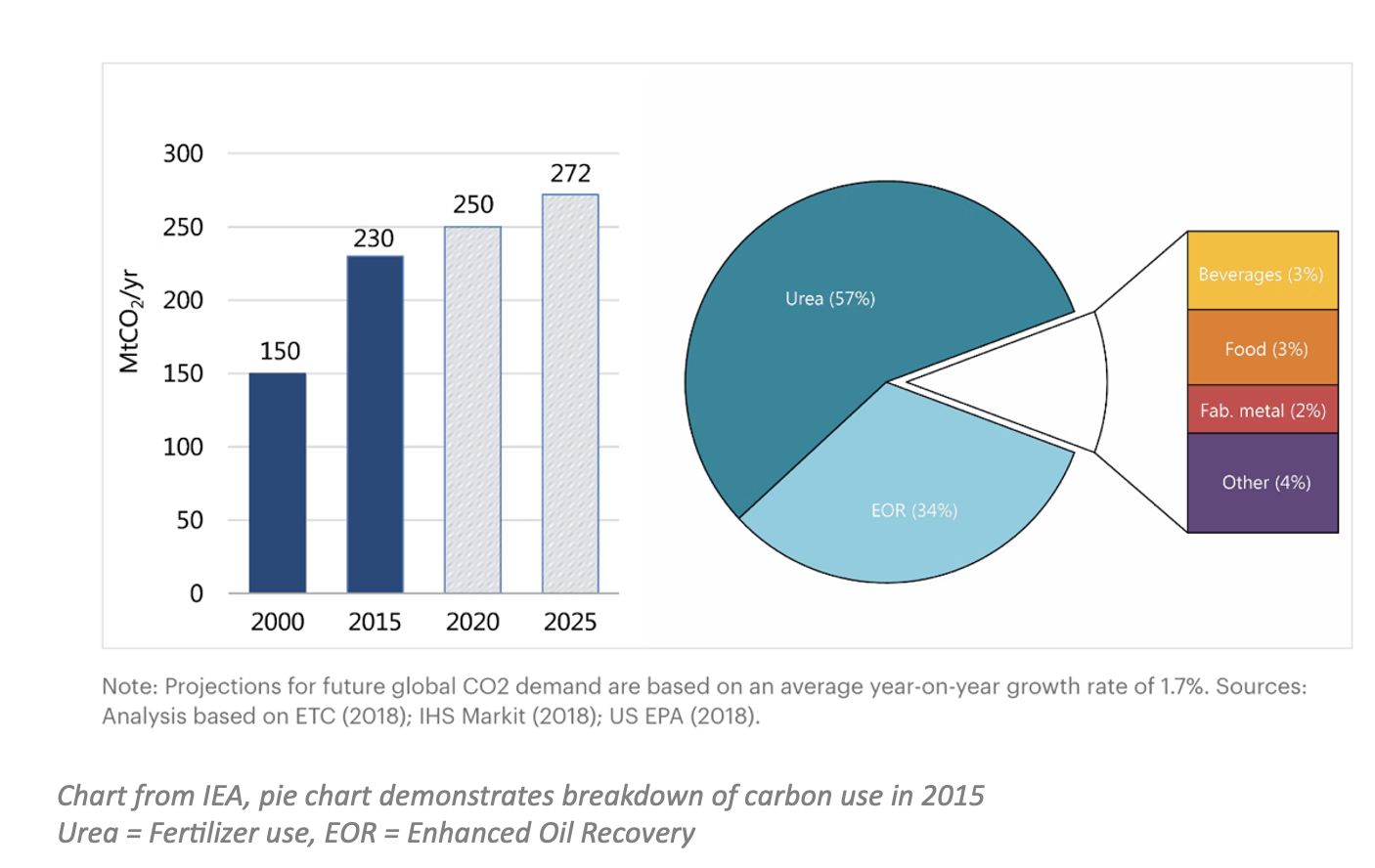
Most of the captured carbon is pumped into storage wells underground. While that is a good way to lock away the carbon, these storage facilities need to be maintained and often occupy a very large area that isn’t used for any other purpose.
Annually, only an estimated 270 million tons/year of CO2 have been used, mostly on fertilisers, food, metal fab, concrete and so on. Some of these uses are problematic, as the CO2 is re-released back into the atmosphere upon use, defeating the purpose of capturing it in the first place. With 270 million tons/year of CO2 used versus 37 billion tons/year of CO2 emitted – we have a very long way to go.
CO2: a useful yet misunderstood element
Earth’s CO2 problem really is about too much of a good thing. Despite its negative climate-related reputation, CO2 primarily is a very useful element and potential resource. Aside from the need for plants to achieve photosynthesis to produce oxygen, everything on earth is carbon-based.
As the Scientific American says, carbon is “the most versatile, most adaptable, most useful element of all. Carbon is the element of life.”
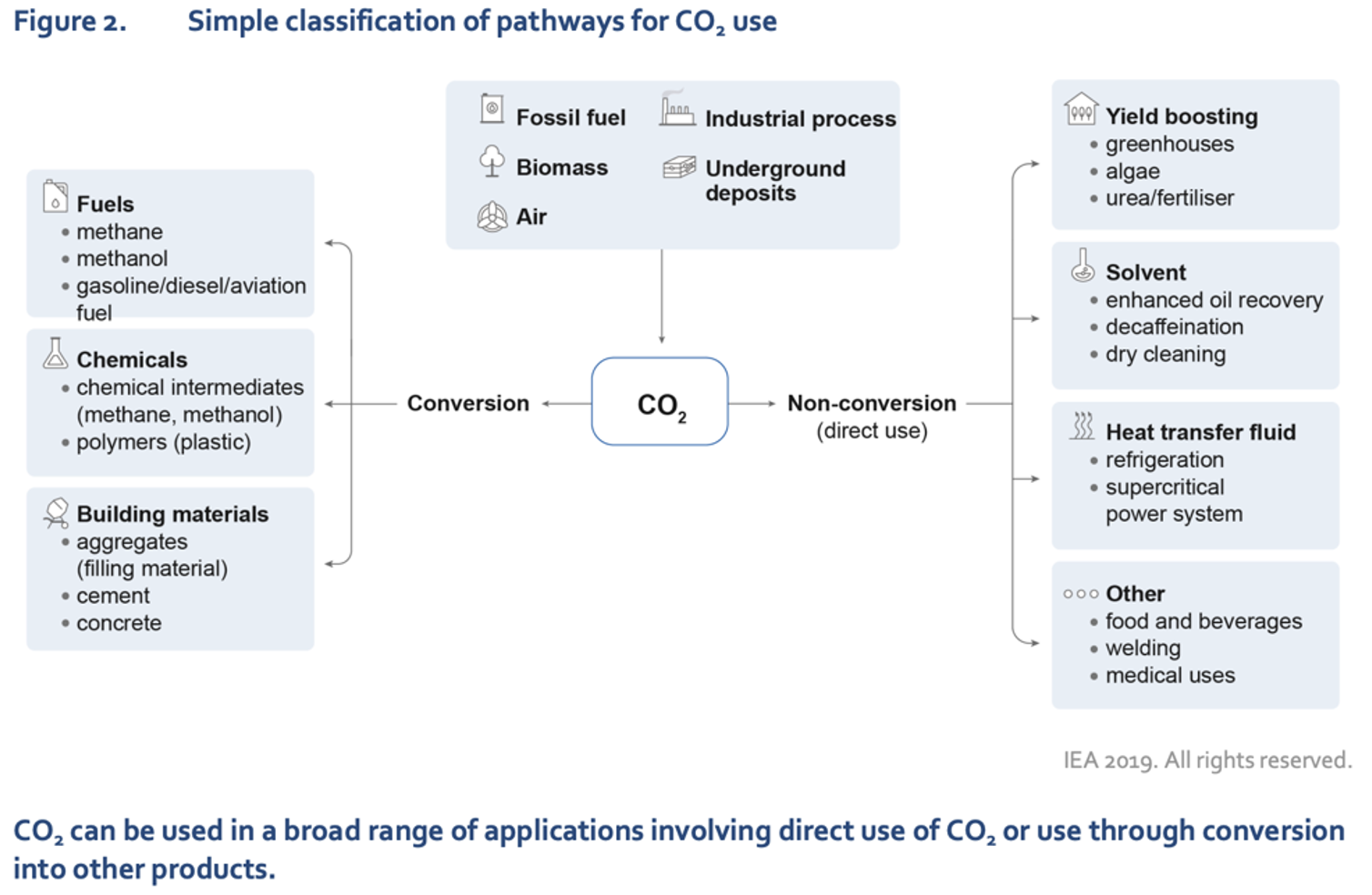
From the chart we can see that carbon, through conversion, can be turned into fuels, chemicals, building materials and so on. Direct uses of carbon include crop yield boosting, use as a heat transfer medium or applications in food, welding, medicines and more. The usefulness of carbon is remarkably broad, with plenty of potential waiting to be untapped.
Carbon has the ability to form stable bonds with many elements, including itself. This property allows carbon to form a huge variety of very large and complex molecules. In fact, there are nearly 10 million carbon-based compounds in living things.
Take for example, the Chimei Asai facility in Taipei, a joint venture of Asahi Kasei Chemicals and Chi Mei Corp. They have been manufacturing around 150,000 tons of polycarbonates per year using CO2 as a starting material for over a decade. (1)
At the price of $15 to $100 a ton, carbon isn’t an expensive resource. Given how affordable it is, we believe more research can be done to use carbon as a resource for practical outcomes.
Carbon utilisation and removal pathways
To understand what we can work with when it comes to handling carbon, we have to understand the different formats of carbon capture: Closed, Cycling and Open Pathway.
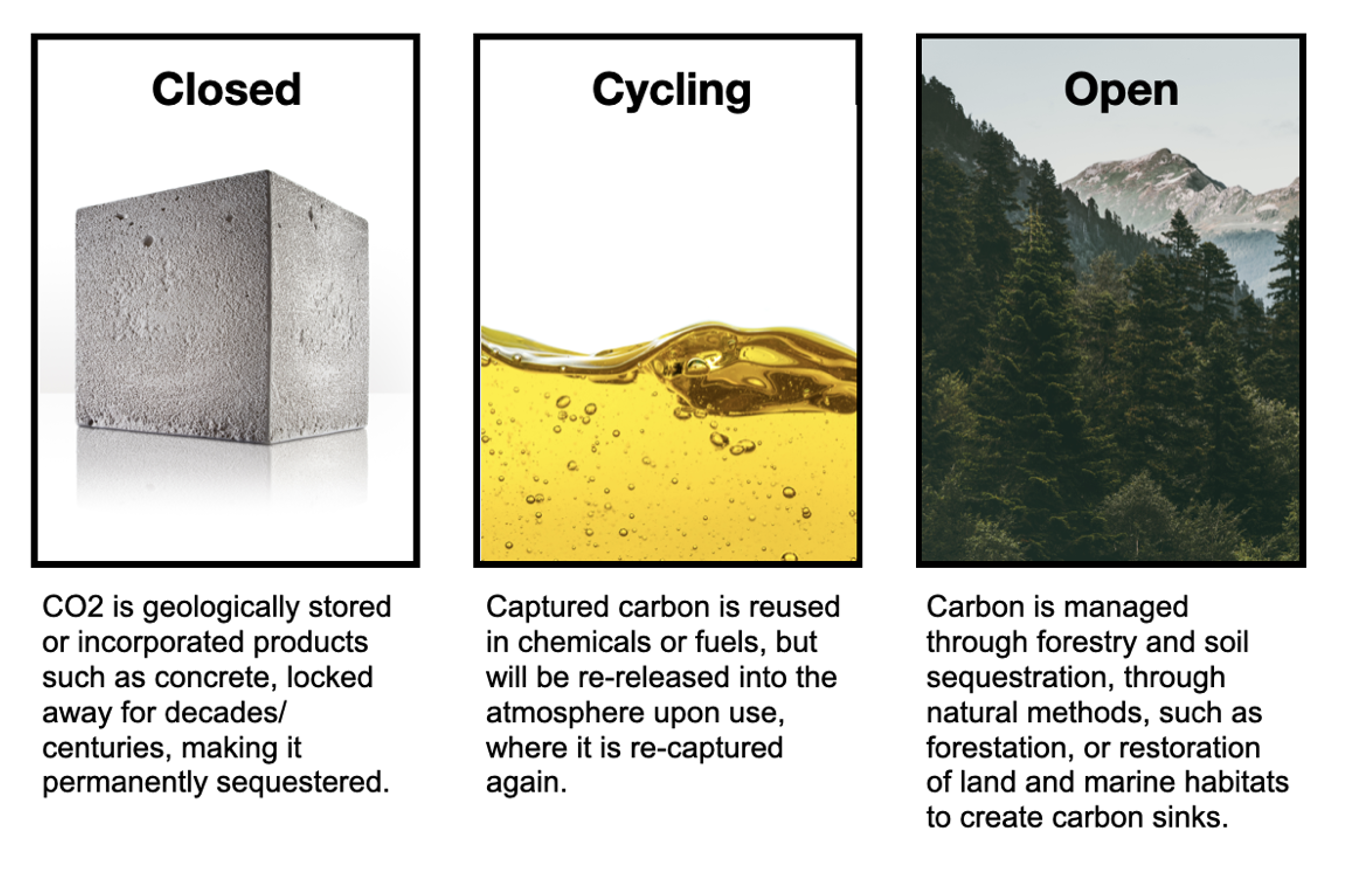
Of the three methods, ‘Closed’ is the most conservative, practical and commonplace. ‘Cycling’ and ‘Open’ have their uses, but both require a large investment in time and resources to make them practical.
There are three methods to use captured carbon:
- CO2 Splitting: CO2 can be split into different components.
- Synthetic Biology: CO2 can be absorbed by microbes/algae to produce chemicals, food or feedstock to make products
- Direct Incorporation: CO2 can be directly incorporated into products.
Splitting carbon to make chemicals and fuels
Electrochemical splitting of CO2 can create useful chemicals, such as syngas, formic acid, oxalic acid, and more. However, as CO2 is an inert molecule, splitting carbon is very complex and expensive. Efficiency rates are also very low, often ranking below 50%. The molecule splitting process also requires plenty of water. If we consider the cost and complexity of the entire process, it’s more efficient to use electrolysis to create hydrogen, a more energy-dense fuel that’s gaining traction globally.
Converting CO2 into liquid fuels that substitute gasoline or diesel fuel only lock in carbon until the fuel is combusted, at which point it is re-released into the atmosphere.
Synbio process to produce fuels, chemicals, food and materials
The synbio route is an interesting one, using genetically modified microorganisms to turn carbon into useful chemicals.
Examples include using microbes to produce chemicals such as bio-ethylene or glucose. Copious amounts of carbon also be used to accelerate algae growth to turn it into an abundant feedstock to make food, biofuels, plastics or even carbon fibre. But the systems are complex, requiring AI-powered photo-bioreactors for biomass production, requiring at least a two-stage dewatering process, and so on.
Generally, the science, research and development behind using synthetic biology to decarbonise our climate is extremely expensive and complex, with many companies exploring this space going under.
Direct incorporation of carbon into things we live with
Direct incorporation is a much more practical method of making use of CO2. Today it’s part of the cement-production process, which ‘locks in’ carbon for much longer. Concrete won’t permanently keep CO2 out of the atmosphere, but can store it for a century or more, which counts as a form of effective carbon storage. The Nature paper calls these “closed” processes.
As the Nature paper says: “Cement requires the use of lime (CaO), which is produced by the calcination of limestone in an emissions-intensive process. As such, unless calcination is paired with carbon capture and sequestration, it is difficult for building-related pathways to deliver reductions in CO2 emissions on a life-cycle basis.”
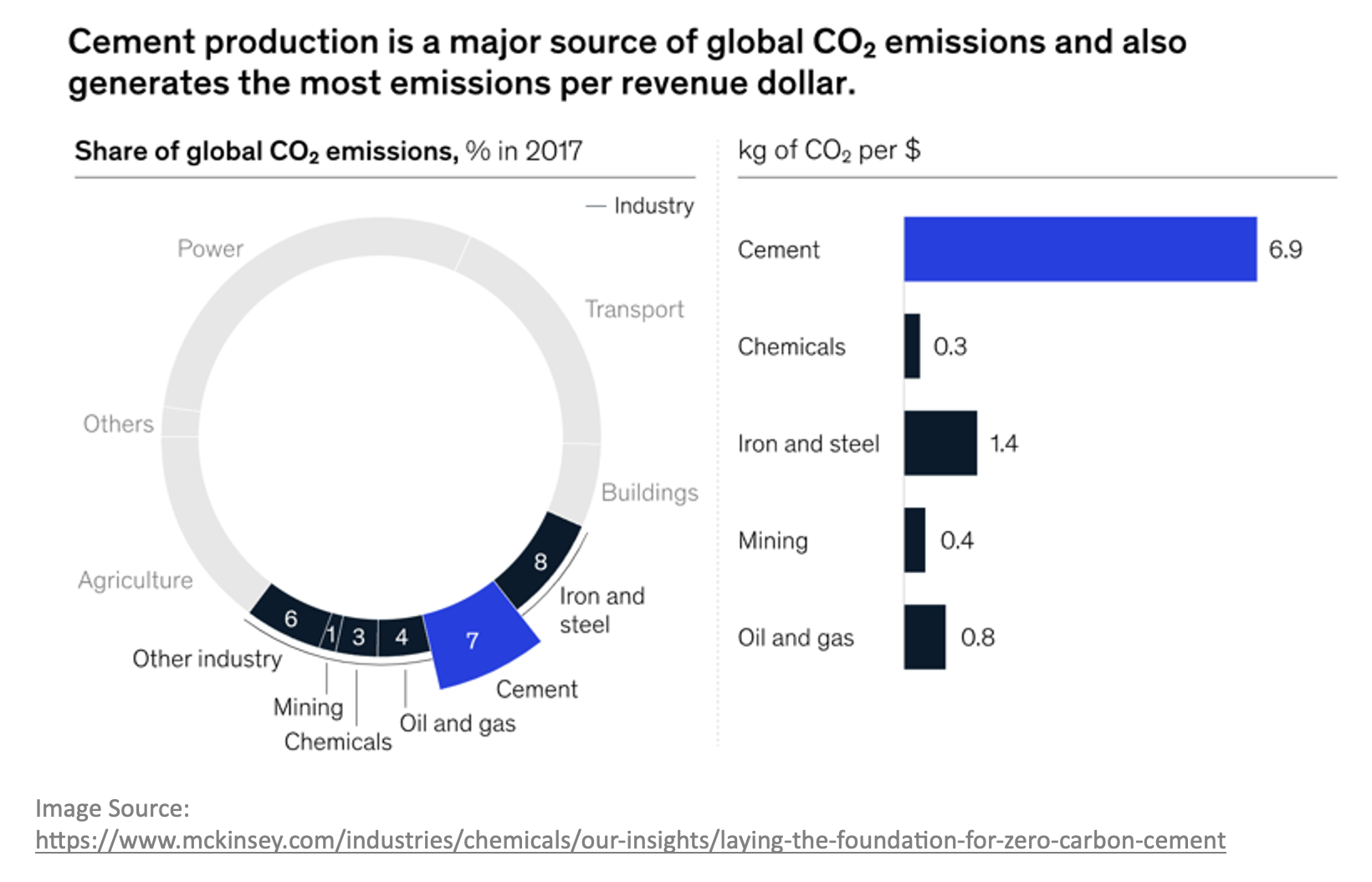
Ironically, cement production itself emits carbon, which needs to be captured and sequestered to make it carbon-neutral. With economies and standards of living around the world improving, and real estate constantly developing, cement production is now the 7th highest emitter of carbon into the atmosphere, with energy production taking number 1 spot. That presents a paradox.
Another common use of captured CO2 is incorporation into fertilisers. However, once the fertiliser is placed in the soil, it’s re-released into the environment within weeks.
So, all that investment and effort in carbon capture and utilisation vaporises in just weeks. Is there any point to that?
Lock carbon into a material that doesn’t degrade: plastic
It’s a challenging goal: make use of abundant captured carbon, don’t let it reintegrate with our climate, and ensure it makes no additional contribution to carbon emissions in the process.
One highly plausible solution we’ve studied over the years, is to incorporate carbon in something that’s very common in our everyday living: plastics.
As the IEA puts it: “CO2-derived products that involve permanent carbon retention, such as building materials, can offer larger emissions reductions than products that ultimately release CO2 to the atmosphere, such as fuels and chemicals.”
More accurately, carbon can be incorporated in polyol, a raw material that’s used to make plastics/PU/surfactants. These become useful materials that we use commonly, and often for a very long time: shoe soles, car seats, insulation, beds, paints and more. When incorporated in plastics, it keeps carbon locked away for long periods, just like carbon in concrete.
As for the production process, making traditional plastics contributes to carbon emissions (though not as much as the concrete-making process). That’s because traditional plastics rely on fossil-based feedstocks. The aim of incorporating captured carbon in plastics is to replace these fossil-based feedstocks, effectively cutting down carbon emissions in the process.
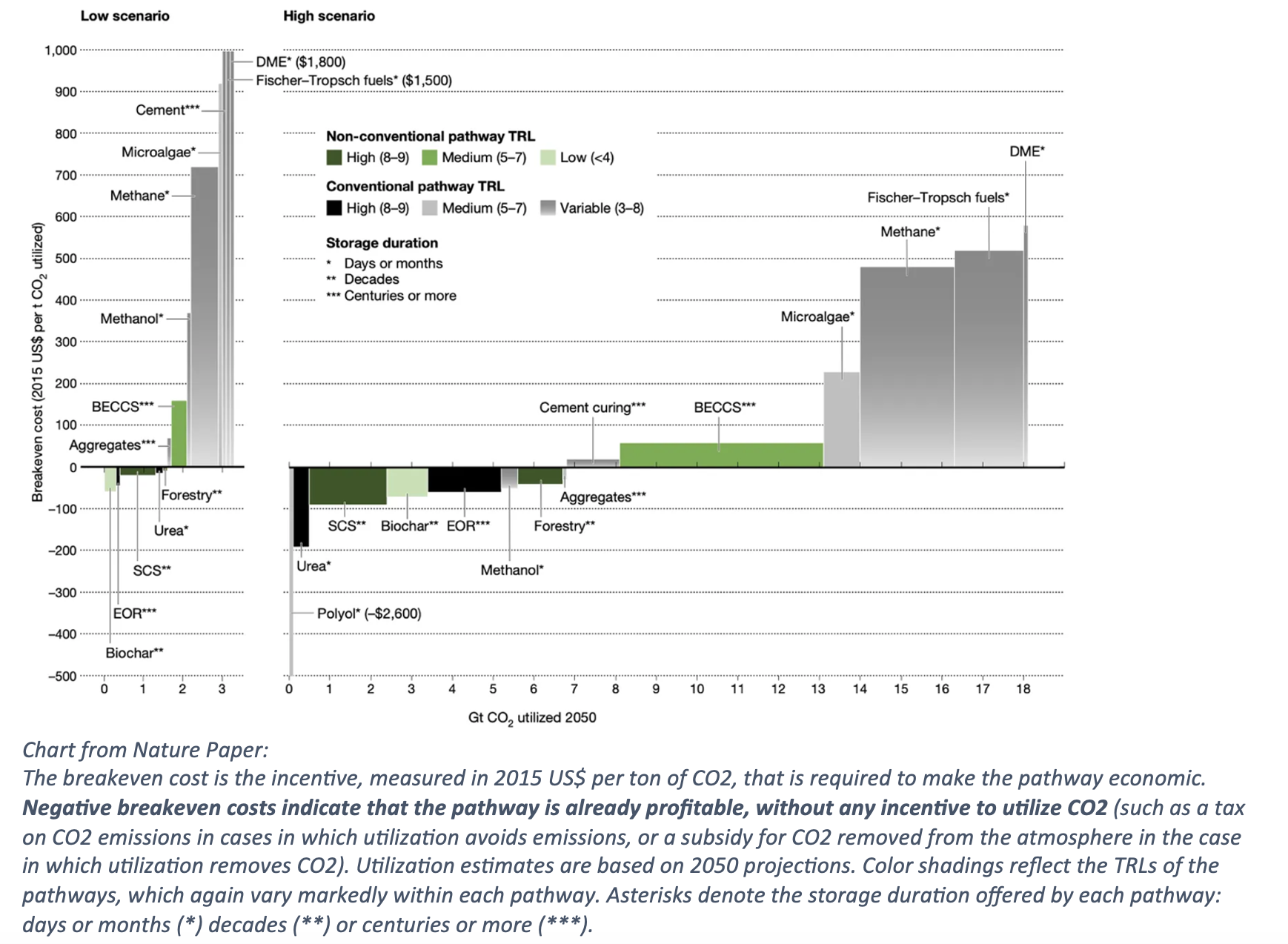
From the chart is very clear the most practical and profitable use of CO2 is incorporation into polyol, a raw material for plastics. A UK startup that’s been developing this method effectively is Econic, which uses a catalyst to incorporate carbon into the PU production process. Best of all, it doesn’t call for additional energy requirements (keeping it carbon-neutral), and it fits seamlessly into existing plastic production processes.
As noted in an IEA paper: Potential climate benefits in polymer production depend on the amount of CO2 that can be absorbed in the material, which can be up to 50% of the polymer’s mass. For example, a polymer containing 20% CO2 by weight shows life cycle CO2 emissions reductions of 15% relative to the conventional production process.
Of course, there are controversies to this: plastics are the very material the world wants to avoid, because it doesn’t degrade well, polluting our seas and environment. Yet, it’s this very fact that helps to lock the carbon away for decades. And plastic isn’t something we can live without – from fundamental living needs to medical applications, it’s a functional, durable and waterproof material that is nearly impossible to replace.
A system in which used plastics made from carbon is responsibly collected/recycled at the end of its use will help mitigate this concern.
Economic considerations: profitability and society
Is CCUS economically viable? If the carbon is mostly stored in geological locations, most probably not. But if captured carbon can become an abundant, key resource that supports our living needs – making buildings from concrete incorporated with carbon, food from carbon, or useful plastic goods made from carbon – it would become very practical. As the human population, cities and needs grow, so do the needs for real estate development, sustenance and functional products made out of plastic. Wouldn’t it be great to have ‘locked-away’ carbon serving our living needs while staying out of the atmosphere?
For now, the CCUS systems in place are very expensive investments with very low or no returns. In part because many haven’t found a practical solution as to what to do with all that captured carbon, or how to lock it in effectively without having it re-released into the environment.
CCUS represents a multifaceted approach to managing carbon emissions. It challenges conventional perceptions of CO2 and opens doors to innovative uses. Though still a subject of debate and development, CCUS is emerging as an indispensable tool for a sustainable future, offering untapped opportunities into a more responsible use of carbon, potentially revealing paths towards both environmental protection and economic benefits.
If we can unlock the potential of captured carbon – making it useful while keeping it out of the atmosphere – the rewards for society, businesses, and mankind could be profound.
(1) Fukuoka, S. et al. (2007), Green and sustainable chemistry in practice: Development and industrialization of a novel process for polycarbonate production from CO2 without using phosgene, Polymer Journal, Vol. 39(2), pp. 91-114, http:// dx.doi.org/DOI: http:// dx.doi.org/10.1295/polymj.PJ2006140.
Dr. Min Zhou is the CEO of CM Venture Capital, a China-headquartered investment company which partners with a number of multinationals to help them invest in cutting-edge hardtech.